Department of Physiology and Biophysics, Keck School of Medicine, University of Southern California, Los Angeles, CA, USA
bergman@usc.edu
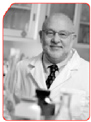
The increase in the prevalence of obesity is well recognized and has been seen not only in North America but in Europe and Asia as well. Associated with increased adiposity is increased risk for a variety of diseases, including diabetes and associated comorbidities, cardiovascular disease, and cancer. Thus, it is clear that if the rate of increase of adiposity could be reduced, there could be important consequences regarding public health.
Key Points
- Visceral (intra-abdominal) fat is very different from subcutaneous fat and is sensitive to adrenergic stimulation of lipolysis.
- The sympathetic nervous system stimulates visceral lipolysis at regular intervals (~11 min.), which increases FFA flux to the liver.
- FFA are likely responsible for the insulin resistance due to visceral fat.
- Increased fat storage results in elevated FFA release at night, suggesting that nocturnal FFA cause the insulin resistance syndrome.
- CB1 inhibition reverses visceral fat storage, liver fat, and insulin resistance, and the effect is due to increased energy expenditure.
The pathogenesis of the so-called "metabolic syndrome" or the closely associated cardiometabolic risk remains less than clear. It is well documented that visceral (intra-abdominal) adiposity in particular is associated with increased risk as well as with insulin resistance [1]. Thus, the relationship between visceral adiposity, insulin resistance, and risk for diseases remains to be explored. Much of the data relating visceral fat to increased risk has been epidemiological, from cross-sectional studies [2, 3]. In our laboratory we have taken an experimental approach, examining the pathogenesis of visceral fat and insulin resistance as well as associated risk factors in the canine model [4]. The latter model allows us to make longitudinal measurements of pathophysiological events and to intervene with appropriate drugs. One such agent is rimonabant, which is an antagonist of the cannabinoid system and limits binding to CB1 receptors.
Similar to human subjects, dogs exhibit a wide range of adiposity in visceral and subcutaneous depots. After fat feeding, we observe a modest weight gain associated with doubling of fat in visceral and subcutaneous depots [5]. The patterns of fat deposition are different between visceral and subcutaneous depots. In the visceral depot we see evidence of progenitor cells that can develop into large adipocytes, and the visceral fat cells are particularly sensitive to lipolysis stimulated by adrenergic agonists. The overall pattern of insulin resistance in this model is related to increases in expression of enzymes that favour lipolysis and enzymes that favour gluconeogenesis. This data supports a model in which excess lipolysis from the visceral fat depot raises free fatty acids (FFA) in the portal circulation, leading to hepatic and peripheral insulin resistance.
The sympathetic nervous system (SNS) also plays a special role: we have found evidence for cyclic stimulation of lipolysis that can be blocked by adrenergic antagonists [6]. These results implicate the SNS in stimulating lipolysis from the visceral fat depot, causing increased portal FFA.
Studies in which we have extirpated all or part of the visceral fat depot in rodents and in the dog model have shown remarkable enhancement of insulin sensitivity. The extirpation studies also support a very important role of visceral fat in pathogenesis of the metabolic syndrome.
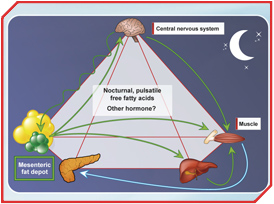
But, if FFA are implicated in causing insulin resistance, why have many laboratories failed to show an increase in levels of FFA under fasting conditions? In our laboratory we took samples over a 24-hour window, before and after feeding of fat. We discovered that there is a powerful increase in FFA levels in the middle of the night (3 a.m. to 6 a.m.) due to increased visceral lipolysis during that period [5]. We hypothesize that it is the outpouring of FFA from the visceral depot in the middle of the night that is responsible for the insulin resistance in the metabolic syndrome (Figure).
We have examined the efficacy of the CB1 inhibitor rimonabant on the development of the metabolic syndrome in the dog model. Feeding of fat to dogs causes continual weight gain; rimonabant stops the gain in weight even in the face of a palatable high fat diet. Rimonabant reverses many of the deleterious effects of fat feeding, including fat deposition, stemming the increase in body weight and increasing energy expenditure. Rimonabant virtually reverses fat deposition in liver, even with increased food fat content. Finally, rimonabant increases energy expenditure, explaining its effects on body weight distribution.
References
- Bergman RN, Kim SP, Hsu IR, et al. Abdominal obesity: role in the pathophysiology of metabolic disease and cardiovascular risk. Am J Med 2007; 120: S3-8.
- Haffner SM. Abdominal adiposity and cardiometabolic risk: do we have all the answers? Am J Med 2007; 120: S10-6.
- Kahn SE, Hull RL and Utzschneider KM. Mechanisms linking obesity to insulin resistance and type 2 diabetes. Nature 2006; 444: 840-6.
- Mittelman SD, Van Citters GW, Kim SP, et al. Longitudinal compensation for fat-induced insulin resistance includes reduced insulin clearance and enhanced beta-cell response. Diabetes 2000; 49: 2116-25.
- Kim SP, Catalano KJ, Hsu IR, et al. Nocturnal free fatty acids are uniquely elevated in the longitudinal development of diet-induced insulin resistance and hyperinsulinemia. Am J Physiol Endocrinol Metab 2007; 292: E1590-8.
- Hucking K, Hamilton-Wessler M, Ellmerer M, et al. Burst-like control of lipolysis by the sympathetic nervous system in vivo. J Clin Invest 2003; 111: 257-64.